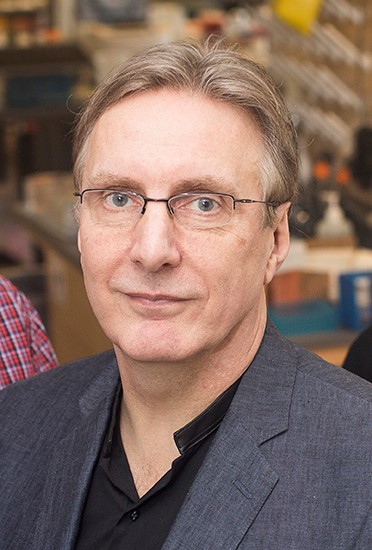
Christopher V. Wright, D. Phil.
Professor of Cell & Developmental Biology
Louise B. McGavock Chair
Director, Program in Developmental Biology
Associate Director, Center for Stem Cell Biology
- : chris.wright@vanderbilt.edu
- :
9415 MRB-IV
2213 Garland Avenue
Nashville, Tennessee - 37232-0494 - : Lab Website
Research Description
Our research aims to gain insight into the molecular and cellular mechanisms coordinating the development of complex organ systems, with relevance to human congenital birth defects and cryptic susceptibilities to disease or dysfunction. The vertebrate body arises by progressively refined decisions. Long-range decisions subdivide the “head to tail” axis, followed by “locking in” of regional tissue territories to form each organ – such as brain, spinal cord, lungs, liver, gut, pancreas – in the right place and right time, and become hooked up to each other, the blood supply, and the central and peripheral nervous systems.
We dissect the transcription-factor–based (TF-based) and epigenetically guided gene-regulatory networks (GRNs) controlling these decisions. A predominant view is that chromatin becomes progressively opened up from a closed-down state, allowing access to genes and their cis-regulatory regions. Gene enhancers may be engaged by pioneer TFs that crack open tightly condensed heterochromatin to make it more open and accessible. Thereafter, additional TFs and regulatory cofactors can be recruited to the newly accessible domains. Cis-regulatory DNA regions (“enhancers”) undergo differential marking by post-translational modifications (PTMs) of histones and other chromatin proteins. They often begin by being marked equivalently by repressive and activating PTMs, yielding a “bivalent” condition. Adding or removing one or the other markings leads to predominant repression or activation by closing chromatin down again or allowing transcription. These processes operate in carefully orchestrated tiers. Some genes allowed to activate by the first-arriving pioneer TFs produce other TFs or regulatory proteins, which “scan” the genome to land on other chromosome locations, thus activating or repressing small or large sets of target genes. Combinatorial action with pioneer TFs is often encountered.
We analyze these processes using bulk or single-cell methods such as ATAC-Seq, single-molecule-FISH (fluorescence in situ hybridization), spatial transcriptomics, epigenetic marking analysis, CUT&RUN or CUT&TAG detection of TF-bound sequences, real-time/time-lapse video- and super-resolution microscopy, 3D tissue-clearing immunolabeling, and many others. Our main recent focus is on how the pancreas forms, and on how genetic-epigenetic mechanisms control lineage decisions. We have worked on this problem in human tissues during the postnatal stages in which major predispositions to type I autoimmune diabetes can emerge. In the mouse, we undertake precision genetic and other perturbational manipulations. Some specific areas are:
- The principal genes involved in acquiring pancreas fate.
- The “run program” that allows an initially tiny number of progenitors to grow into a much larger organ with precisely arranged endocrine and exocrine cells.
- Endocrine-cell birth in the pancreas from a dynamic, relatively short-lived epithelial niche.
- Generation of endocrine cells from lineage-primed mitotic progenitors, which in turn form post-mitotic cells that loosen from the epithelium to cluster into islets of Langerhans.
- Human TF mutations that cause endocrine-cell disturbance and permanent neonatal diabetes.
- Cryptic epigenetic mis-programming. For example, deficiency in the Mnx1 TF – selectively expressed in insulin-producing beta cells – does not block progress down an apparently normal beta-cell program with “on-course momentum”. In the early postnatal period, however, an unknown intra- or extra-cellular signal causes GRN rewiring and results in a sudden, profound shift into somatostatin-producing cells.
* * *
Vanderbilt Faculty Profile
Google Scholar
Publications