The laboratory of Ege Kavalali, professor and chair of the Department of Pharmacology, published a new paper in Nature Communications that determined that liquid-liquid phase separation plays a key role within the nanostructure of synapses, and that its disruption affects evoked but not spontaneous neurotransmission.

Since the neuronal cytoplasm is aqueous, distinct liquid phases can form spontaneously within it that are defined by different concentrations of macromolecules. In LLPS, liquid condensates—or membrane-less organelles—that are enriched in specific protein-protein or protein–nucleic acid complexes separate from a de-enriched, dilute phase, creating distinct liquid phases within the cytosol. In principle, this is similar to mixing oil and water—despite both being liquids, they still separate into distinct phases.
We sat down with the paper’s first author, Natalie J. Guzikowski, a Ph.D. student in the Kavalali lab, to learn more about their recent work.
What issue/problem does your research address?
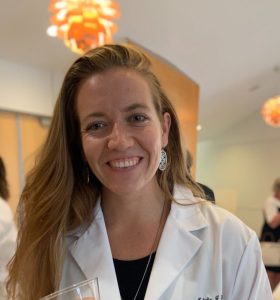
Over the past decade, a growing amount of research has revealed how liquid-liquid phase separation mediates protein interactions throughout the cell and, furthermore, at the synapse. While this work has been exciting, it has been hard to understand the physiological role these liquid condensates play within the context of a firing neuron. Our research investigates how these liquid-like properties contribute to the main function of the neuron in synaptic signaling.
The synapse, a highly ordered and dynamic subcellular system, maintains autonomy, organization, and high-fidelity neurotransmission without a bounding membrane. Unlike other cellular sub-structures (for example, the nucleus, mitochondria, and endoplasmic reticulum), the synapse does not possess an enclosing membrane to aid in compartmentalization. Despite this, the synapse is still able to orchestrate numerous, distinct functional pathways fundamental in larger-scale brain circuits and higher-order processing. What physicochemical and structural properties enable this?
What were your top three findings?
We found the conservation of fundamental principles from liquid condensate biology in the nano-organization of the synapse, demonstrating how a multitude of protein interactions work in synchrony to compartmentalize the synapse into distinct signaling zones
We also found that the organization of the presynaptic milieu, including both synaptic vesicle pools and active zone scaffolding complexes, is fluid in nature, and dynamic liquid condensates are essential for efficient and precise neurotransmission.
Finally, we learned that LLPS is necessary within the nanodomain of the synapse and that the disruption of this nano-organization largely spares spontaneous neurotransmission, revealing the specificity of LLPS in directing evoked release.
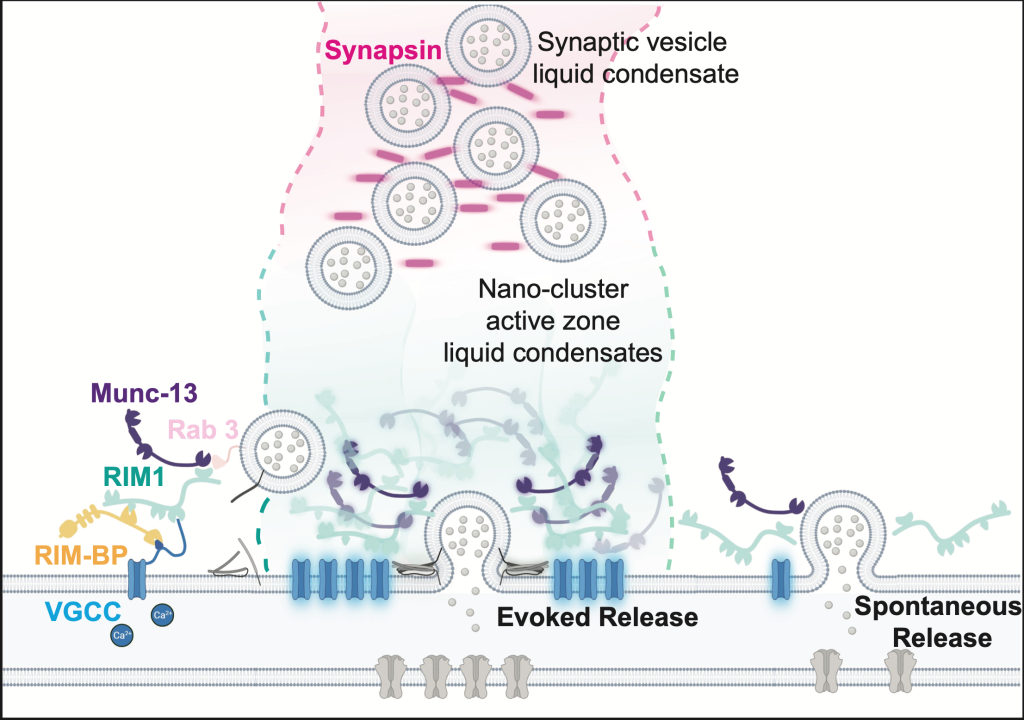
What are spontaneous and evoked neurotransmission?
Action potential–dependent or evoked neurotransmission is the mode of neurotransmitter release people are more familiar with, in which an electrical signal triggers the release of neurotransmitters at a synapse, which in turn triggers a new electrical signal in the proceeding neuron, and so on. However, neurotransmitter release can also occur spontaneously, in an action potential–independent manner that is fundamental in homeostatic plasticity and development. Spontaneous neurotransmission is also a substrate for psychiatric disease intervention.
What was unique about your approach to the research?
One aspect that makes our research unique was that our manipulation of LLPS complexes was independent of targeted structured protein domain interactions, protein trafficking, and genetic perturbations, which helped establish the nanospecificity and physiological relevance of LLPS at the synapse.
Our work also investigated LLPS through a different lens than other LLPS studies, as we used electrophysiology to understand—in real time—how the disruption of LLPS effects neurotransmission, revealing a high degree of specifically in how exactly LLPS works.
What are the long-term benefits of this research?
Further demarcation of the functional specificity of LLPS complexes will be instrumental in understanding the nano-environment of the synapse, which is essential to uncovering mechanisms underlying neurological diseases and their treatment.
Where is this research taking you next?
Our work is bridging the gap between liquid condensates identified in vitro and the functional implication of LLPS at the synapse. We hope other researchers build on our work by continuing to investigate synaptic physiology at the intersection of structure and function.
Go deeper
The paper “Functional specificity of liquid-liquid phase separation at the synapse” was published in Nature Communications in November 2024.
Funding
This research used funds from the National Institute of Mental Health.