By Natalya Ortolano
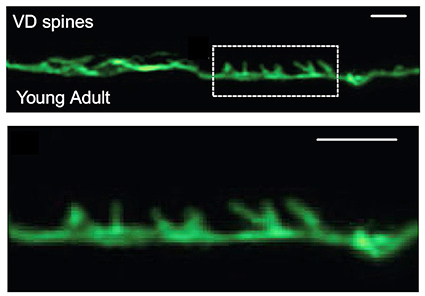
When Nobel laureate Santiago Ramón y Cajal started staining bird brains in the late 1800s, he ignored the spine-like protrusions decorating the neurons. He wasn’t the first to notice these protrusions; dogma dictated that they were a side effect of staining brains. However, he became convinced these protrusions were real. He likened these structures to the thorns on a rose, naming them espinas or spines. Since Ramón y Cajal’s landmark publication describing dendritic spines in 1888, they have been the focus of much research in neuroscience.
It was long thought that dendritic spines were only present in vertebrates. Recent studies show that invertebrates do have dendritic spines, but it had never been demonstrated that the nematode Caenorhabditis elegans had spines. A publication from the laboratory of David Miller (Cell and Developmental Biology) published in eLife demonstrates that the C. elegans has neurons decorated with spines that function similarly to those in mammals.
Just like with Ramón y Cajal, the discovery of dendritic spines in C. elegans began with an unexpected observation.
Andrea Cuentas-Condori, a graduate student in the Miller laboratory, studies actin’s role in the formation of synapses, structures that pass messages between neurons through electrical or chemical signals. Actin works with a network of proteins to form the cytoskeleton, an arrangement of filaments that regulate cell morphology. The actin cytoskeleton plays a known role in the morphological changes that occur in a synapse when it’s relaying a message.
To explore the role of actin in synapse formation, Cuentas-Condori expressed a shortened, fluorescent form of an actin marker in a particular C. elegans neuron. C. elegans are transparent, which allows for live imaging of neurons and dendritic spines. When she imaged the neuron, she was surprised to see that it had spine-like protrusions. Unknowingly echoing Ramón y Cajal, she initially ignored it. Yet after seeing them several times, she suspected that these protrusions were dendritic spines, but she couldn’t find any previous reports of them in C. elegans.
Modern research has shown that dendritic spines are important for learning and memory in vertebrates. Dendritic spines are part of the synapse and are present at the dendrite, the part of the neuron that receives signals sent through the synapse. Dendritic spines play an essential role in signal transmission between neurons, and issues with forming and maintaining these structures can lead to human diseases like schizophrenia or Alzheimer’s disease.
“Since spines have been correlated with consciousness and learning processes, it was previously thought that invertebrates did not have these structures,” Cuentas-Condori said.
Cuentas-Condori followed her gut and set out to determine if these protrusions shared key features with mammalian dendritic spines.
Using quantitative, live microscopy, Cuentas-Condori showed that, similarly to vertebrate spines, invertebrate spines were more enriched with actin than other parts of the neuron. The spines had a thin, elongated morphology, like mammalian spines, and relied on a dynamic actin cytoskeleton to change their shape.
Additionally, she showed that dendritic spine density and morphology are regulated during development by synaptic signaling, just like in vertebrate neurons. Spine density increases as C. elegans age due to extended signaling at the dendrite; however, when signaling was infrequent or shortened, spines were sparser.
Cuentas-Condori also demonstrated that invertebrate dendritic spines are functional. She showed that C. elegans’ spines have oscillating levels of calcium, a key synaptic signal, indicating that they play a vital role in neuronal communication at the dendrite as seen in mammalian spines.
The research showed, for the first time, that dendritic spines are not only present in C. elegans, but that they have the same morphology, development, and function as mammalian spines. This opens the door to using models like C. elegans in novel ways, like probing the role of dendritic spines in memory and learning. In fact, Cuentas-Condori thinks these structures do much more.
“I think the spines help the cell reach out to its target. The dendrite is so far away from its target, it needs to protrude to reach the signal,” she said. This basic function is why Cuentas-Condori thinks these structures are evolutionarily conserved.
The Miller laboratory is currently working to further characterize these spiny neurons. Future studies in C. eleganscould expand our understanding of how dendritic spines develop and are maintained, providing critical insight into how these processes work in our own brains, and how they go awry in diseases like schizophrenia and Alzheimer’s.