By Marissa Shapiro
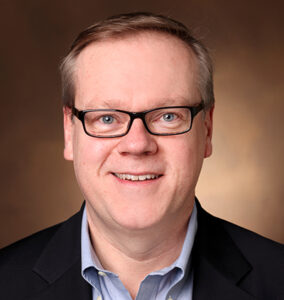
The human genome is a rich and diverse field of study at which Vanderbilt University and Vanderbilt University Medical Center scientists stand at the forefront. William Tansey, professor of cell and developmental biology and biochemistry and co-leader of the Vanderbilt-Ingram Cancer Center Genome Maintenance Program, gives us a bird’s-eye view of the field and some of Vanderbilt’s research contributions to the space.
What is the discipline of epigenetics and human genome regulation?
In general, the big question that scientists are trying to answer is how genetic information— our DNA and the information encoded in DNA— is used. The basic problem is that every cell in your body has, for the most part, the same DNA content. And we know DNA is the blueprint for life. But we also know that all the cells in our bodies are different: A cell in the iris of our eye is very different from a cell in our liver or a cell from muscle. How can the same blueprint lead to such differences?
For a long time, scientists have been asking questions about how cells achieve such diversity and plasticity even though they’re working from the same script. The answer to that question is that cells read different parts of the blueprint depending on what their function is—and the process of reading the blueprint, and different bits of it, is called “gene expression.”
The field of gene expression is trying to understand—in very broad terms—the molecular processes that allow the use of the genetic information. Some of the control of gene expression is hard-wired into the DNA sequence. But not all. This is where epigenetics comes in.
Formally speaking, epigenetics—“epi” meaning “above”—is any form of control of gene expression that is above the level of the DNA or that is not encoded directly in the DNA.
It refers to a layer of regulation that has to do with how the genetic material is labeled and packaged. Every cell has about two meters (six feet) of DNA in it, and it exists in huge strands that are folded and wrapped around proteins into structures that layer on top of each other and pack into a sphere that’s just a few micrometers in diameter. It’s the ultimate storage solution on one hand, but it also means that the cell can control how information is used by packaging or labeling the genetic material in specific ways.
Control of gene expression by this level of packaging and labeling is epigenetics. At a molecular level, it is driven by chemical alterations in the DNA and those proteins it wraps around. Although these chemical alterations don’t change the sequence of the DNA, they can very much determine what part of our genome is expressed in a given cell.
How does this fit in the blueprint analogy?
Epigenetics is like someone taking a pencil and crossing something out—or adding something—on a blueprint, saying, “don’t make that here, make it over there.” And that’s a good analogy because, unlike the messages encoded in our DNA sequence, the messages that epigenetics communicate are reversible and changeable. That allows adaptability; genes can be expressed in specific places, at specific times, and in response to specific signals or cues.
How do scientists like yourself work to understand the human genome through epigenetics and gene expression?
The genome, epigenetics, and gene expression are all interconnected because, ultimately, gene expression is all about anything that will impact or control the ability of a cell to express a piece of its genome. Historically, the fields of gene expression and epigenetics were somewhat separate because the mechanisms of epigenetics were not really understood. But in the last two to three decades, that all changed. Now, when we talk about a piece of DNA and how that gene is regulated, we have to figure out if it’s regulated through DNA sequence, epigenetics, or a combination of both. Usually, it ends up being both. This field of gene expression is massive— it’s one of the oldest fields in molecular biology. Everybody bites off a little piece of the problem. I, for example, study how gene expression goes wrong in cancer by looking at a protein called MYC (“mick”), which alters gene expression and is influenced by epigenetic processes. My interest is in understanding how MYC controls gene expression and using that information to think of ways to stop MYC in cancer. But that’s just one sliver of the gene expression and epigenetics fields, and it’s just a fraction of gene expression research in Basic Sciences.
How are scientists in Basic Sciences probing gene expression and epigenetics?
We have a number of superb researchers here who work on different aspects of gene expression and epigenetics! My familiarity with our research in this area is mostly from the cancer perspective because I co-lead the Genome Maintenance Research Program, a cancer-focused program made up of 25 investigators working on understanding all aspects of how the genome is packaged, expressed, duplicated, and maintained— and how this can go awry in cancer.
We have amazing strength in this area. And we are attacking the problem from different perspectives. The primary interest of Emily Hodges, for example, is understanding DNA methylation, a small chemical modification that can be added to DNA that acts epigenetically to regulate gene activity. Scott Hiebert is also interested in gene regulation and epigenetics from the point of view of how aberrant gene expression occurs in leukemias. Jared Nordman and David Cortez, my GMRP co-leader, work on how epigenetics intersects with DNA replication and repair and maintenance of DNA integrity.